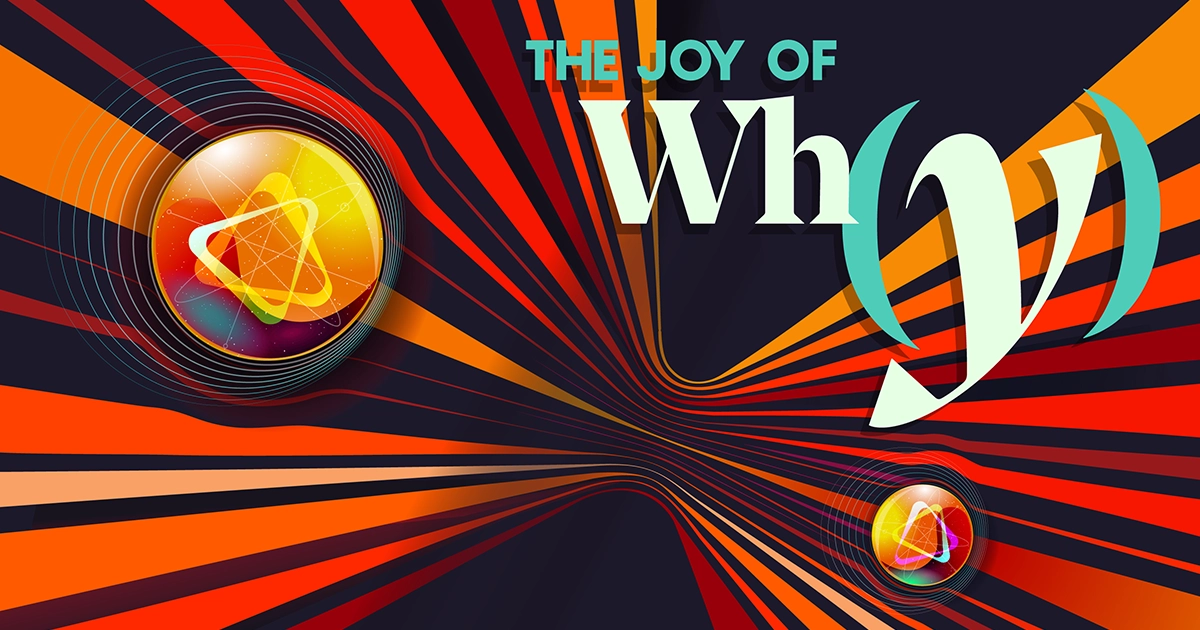
Introduction
Quantum teleportation isn’t just science fiction; it’s entirely real and happening in laboratories today. But teleporting quantum particles and information is a far cry from beaming people through space. In some ways, it’s even more astonishing.
John Preskill, a theoretical physicist at the California Institute of Technology, is one of the leading theoreticians of quantum computing and information. In this episode, co-host Janna Levin interviews him about entanglement, teleporting bits from coast to coast, and the revolutionary promise of quantum technology.
Listen on Apple Podcasts, Spotify, Google Podcasts, TuneIn or your favorite podcasting app, or you can stream it from Quanta.
Transcript
JANNA LEVIN: When I say the word teleportation, what comes to mind? Maybe it’s the transporter from Star Trek instantly beaming the crew down to a planet, or the time-traveling TARDIS of Doctor Who. In science fiction, teleportation is an expedient device to deliver people from one place to another with no time wasted on the journey.
But quantum teleportation? Well, that’s something dramatically different — and entirely real.
I’m Janna Levin and this is “The Joy of Why,” a podcast from Quanta Magazine, where I take turns at the mic with my co-host, Steve Strogatz, exploring some of the biggest questions in math and science today.
Quantum teleportation is the power to disappear from one location and appear at another, without traveling in between. Though we may never match the movies, the technology will likely revolutionize communications, computing and our understanding of the world around us.
Today, we’re joined by one of the leading experts on quantum teleportation. John Preskill is a professor of theoretical physics at the California Institute of Technology and the founder and current leadership chair of the Institute for Quantum Information and Matter. His research has explored particle physics, quantum field theory, and quantum aspects of the early universe and black holes. His current work applies this research to intractable problems in quantum computing and information. John, welcome to “The Joy of Why.”
JOHN PRESKILL: Glad to be here, Janna.
LEVIN: Glad to have you. I want to get into the details of this incredibly technical subject, but can you start us off with one of the core concepts, which is the idea of entanglement, quantum entanglement?
PRESKILL: Well, entanglement is the word we use for the characteristic correlations between parts of a quantum system.
First of all, what do we mean by a correlation? We can talk about correlations for ordinary bits. Let’s say that you have a bit, which is either 0 or 1. And I have a bit, which is either 0 or 1. Then if we both have 0 or we both have 1, that’s a correlation between our bits.
In the case of qubits, they can be correlated in a similar way. When we observe or measure the qubit — the quantum analog of a bit — we acquire a bit. But what’s different about the quantum case is that there’s more than one way to look at a qubit.
So you can think of it as a box that has a bit inside. Inside there’s either a 0 or a 1. And I have two ways of looking inside the box. It’s got two doors. I can either open Door #1 or I can open Door #2. And each way, I see a bit.
And we could have a correlation for both ways. If we both open Door #1, we see some correlation between the bit that you acquire and the bit I acquire. And if we both open Door #2, we see a correlation, which in general could be different.
And it’s because we have these multiple complementary ways of looking at a qubit that they have correlations that are more interesting and complex than the correlations among ordinary bits.
But the mystery is this: You can’t observe a qubit without disturbing it. This is a very important difference between ordinary information and quantum information.
LEVIN: So let’s say I disturb my particle and force it to assume a definite state. We can call that a measurement process, or maybe I do it by accident. And I find out it’s a 0. And it was correlated with your particle in such a way. Does that truly — as people say — faster than the speed of light impose on your particle that it assume a certain state in order to respect the correlation?
PRESKILL: No, sadly, it does not. Oh, I wish it did. If I look at my qubit, it doesn’t matter whether you’ve looked at yours or not. I’m just going to see a random bit. So, it’s only after we both look and we talk to one another that we can tell that we had a correlation.
But, unless we talk, each one of us is just going to observe pure randomness, but with an equal chance of being 0 or 1, and there’s no way that can convey any information.
LEVIN: Now, of course, if we do discuss with each other, that has to travel slower than the speed of light, that part of the communication.
PRESKILL: Well, you can get pretty close to the speed of light, but no faster. So that’s, that’s a big issue, that we really can’t, even if we have entanglement, send information from me to you faster than the time it takes light to travel from me to you. Entanglement does not change that story.
LEVIN: Amazing. Now, here we’ve discussed entanglement, which dates back to thought experiments that [Albert] Einstein was doing to try to wrestle with, and sometimes against, quantum mechanics. Now, why did Einstein famously refer to this as “spooky action at a distance“? Or sometimes the translation is “ghostly action at a distance.”
PRESKILL: Well, Einstein felt very strongly that there should be no randomness in the fundamental laws of physics. He felt that if we know everything that can be known — that the laws of physics will allow us to know — about a physical system, then we should be able to perfectly predict what we’ll see when we observe that system.
And entanglement does not obey that principle. There really is true randomness in the world. Even if we know everything about that entangled pair of qubits that you and I share, still you are powerless to predict what you see when you look at that qubit. It’s just a random bit. And it’s not because you don’t know. It’s that it cannot be known.
LEVIN: How does this become an important lever in quantum teleportation? That in and of itself is not quantum teleportation. So, how is it exploited?
PRESKILL: It’s a subtle question. So let’s talk now about what quantum teleportation is.
LEVIN: Please, yes.
PRESKILL: So you’re in New York now, right?
LEVIN: I’m in New York, yeah.
PRESKILL: All right, Janna, I’m currently in California, and you are in New York, and I happen to have here in California a qubit. It’s right here in my hand. It’s encoded in a little atom. But a quantum FedEx makes mistakes sometimes, so they sent me this qubit, but it was intended for you. OK? So, somehow I have to figure out how to get my qubit over to you. And if we had some conduit that we could use to send the atom from California to New York, that would be one way of getting the qubit over to you. But we don’t have any such connection that I can use to send atoms.
But you don’t want the atom, you want the information that’s in the atom. Well, it so happens that you and I cleverly had the foresight to create a pair of entangled qubits yesterday, anticipating that we might be able to make use of them at some point.
And here’s what I can do. I can take this qubit that I received today. I don’t know what information is in it. It’s some qubit that was delivered to me. And I can observe it together with my half of the entangled pair of qubits that you and I share.
And now, I’m observing two qubits, and I do it in a — let’s call it an entangled measurement. We look at the two collectively, and I can get two bits of information from observing them. And then — now, over an ordinary communication link, like what we’re using now — I can send those two bits of information to you. And then, you can use those two bits of information to perform an operation on your qubit in New York.
And now, that qubit in New York has all the same quantum information as that mystery qubit, which I received today. I don’t know what the state of that qubit is, and in fact, I destroy it in my lab when I observe it. But we’re able to “reincarnate” it, so to speak, in New York. And you only need those two bits of information in order to reconstruct that qubit perfectly. That’s quantum teleportation.
LEVIN: So, in some sense, you had a quantum state in California that you wanted me to be able to reproduce in New York without sending it via FedEx, driving across the country. You wanted me to be able to do it without physically moving anything in between. So you figured out this clever way for me to reconstruct the state in my own lab with just those simple instructions.
And in that sense, it teleported. It disappeared on your end because you destroyed the state, and the process of trying to find the information you needed to convey to me. But it reappeared in my lab once you did convey the information. Did I miss something crucial in that paraphrasing?
PRESKILL: Well, I think there are a few things to amplify in what you said. First of all, I don’t quite agree with your statement that I didn’t send anything physical to you. In fact, I did. I sent you two bits of information.
LEVIN: Oh, you did send me information across the internet.
PRESKILL: I can’t do that without sending something physical.
LEVIN: Agreed.
PRESKILL: Maybe it was photons, which went through an optical fiber from California to New York. And that communication between us was actually necessary for this to work.
But it’s not enough. It’s a funny thing about qubits. If I want to prepare a state of a qubit, I need a lot of information. You can sort of geometrically visualize a qubit as like a little arrow pointing in a three-dimensional space. You know, like the surface of the Earth. And if I want to tell you how I prepared the qubit, I’m picking a point on that globe, so I have to give you the latitude and the longitude to very high precision to tell you precisely how that qubit was prepared.
So, in a sense, there’s a lot of information that goes in, but very little comes out, because when you observe it, you just get one bit. So that one bit isn’t going to tell you how to put the qubit, so to speak, on the globe at some definite latitude and longitude. So that’s why teleportation is remarkable, because I only sent you those two bits, and that was enough for you to reconstruct it perfectly.
It’s the two bits together with the entanglement that we shared, which we had the foresight to prepare yesterday.
LEVIN: Right, so that’s a big difference. That’s amazing, now. You are sending me information physically, either internet or light signals or however you’re sending them to me. But somehow, I’m getting more information because of the entangled setup that we agreed on.
So it’s not as though you had your IKEA desk, and I needed some information on how to build mine and you smashed yours to pieces to figure out how it was assembled. You’d still have to tell me every little bit of information. So there’s something fundamentally different about the quantum process from the classical process. What’s the advantage of that? Why is it so exciting? What’s the big deal?
PRESKILL: Well, first of all, Janna, you and I are theoretical physicists, so, you know, it doesn’t take much to get us excited.
LEVIN: [laughing] Absolutely.
PRESKILL: But what is it useful for? That’s a good question. So, let’s suppose we want to distribute entanglement around the world. It sounds pretty cool right? We took for granted that you and I could share entanglement between California and New York, and we didn’t talk about how we managed to do that.
In fact, we don’t know how to do that right now with the technology that currently exists. There’s no reason why we can’t in principle, but for practical reasons, with the technology we currently have, we can’t send a qubit from California to New York and have it arrive undamaged.
The best way we have to send qubits is by sending photons through optical fiber, and optical fiber has losses. So if you try to send a qubit a hundred kilometers, it has only about one chance in 50 of making it without disappearing. And if I tried to send it a thousand kilometers, which still isn’t enough to get to New York, there’s almost zero probability it’ll make it.
So, how can we share entanglement? Well, we think we’re going to do it by using teleportation. It sounds a little circular, right? Because we need entanglement to do teleportation. But here’s the idea: I can send a qubit, say, 10 kilometers, you know, or 50 kilometers, with a pretty high probability of success.
LEVIN: That’s still pretty good.
PRESKILL: Yeah, that’s not too bad. But now let’s suppose I want to get all the way from California to New York, so what I do is, I introduce lots of little nodes along the way, where we’re going to sort of connect the quantum communication. So let’s imagine we’re trying to get from A to C and what we do is we share entanglement between A and B and between B and C. And then we have a way of making a measurement at B of the two halves of these entangled pairs. We call it entanglement swapping.
You do a measurement of the two qubits at B, and then you tell A and C, “Oh, here’s the measurement result I had.” Now A and C can share entanglement. OK? In effect, we’re extending the, um, the range of the entanglement. It’s a variant on teleportation.
And I haven’t told you the whole story yet, because if the entanglement from A to B isn’t so good and the entanglement from B to C isn’t so good, we can take lots of entanglement pairs that are kind of noisy and imperfect, and there’s a way to distill them down to fewer entangled pairs, which are much higher quality. And by doing that repeatedly, we can make a connection between California and New York, and then we can use it for whatever we want. We might use it to develop that shared key that we know is private, or we might use it to send quantum information.
Here’s a more mundane, shorter-distance way in which we can use teleportation. If we have two chips in a quantum computer, and we want to send quantum information from one to the other, the way we can do it is by establishing entanglement between the two chips, and then using teleportation to send information from one to the other. And that’s probably going to be essential for scaling up quantum computing to large systems that can solve really hard problems.
LEVIN: We’ll be right back.
[Break for ad insertion]
LEVIN: Welcome back to “The Joy of Why.”
So you really are talking about technologies. I’m aware that you recently did some groundbreaking for a new center at Caltech. The Center for Quantum Precision Measurement, I believe it’s going to be called.
PRESKILL: That’s correct, yeah. You’ve been doing your research.
LEVIN: Yeah. And is that geared partly towards advancing technologies? As you said, you are a theoretical physicist. This is what some people have said, the “surprising usefulness of useless ideas.” But are you geared towards advancing technologies with a center like that, or are you looking to revolutionize, really, our fundamental understanding of quantum mechanics, or both?
PRESKILL: We can’t really separate those things. Science and technology advance together. As our science gets more sophisticated, we develop better technologies, and that enables new discoveries. When science advances, it’s through a combination of new ideas and new technologies.
So, I’m interested in quantum computers, for example, and there are reasons to expect that eventually that’ll have a big practical impact on society. But it’s also a wonderful instrument for scientific discovery. So at the Center for Quantum Precision Measurement, yes, we’ll be developing technology, but with an eye on better measurement strategies that exploit properties like quantum entanglement, which will allow us to measure things with greater precision and less invasiveness.
Everybody wants to measure things better, and quantum strategies can help us to do measurements that wouldn’t otherwise be possible. That’s really the intellectual theme of that center.
LEVIN: Yeah, and everyone wants to control information better, faster.
PRESKILL: Well, everybody understands information is important, and what quantum information will be used for and where the big practical impact will be — there’s still a lot of open questions about that.
But we can anticipate that with quantum information, with quantum computing, using quantum entanglement for measurement, we’ll be able to do things that we couldn’t do before. And that’s going to have a practical impact eventually.
LEVIN: Do you foresee that practical impact extending to our everyday lives?
PRESKILL: Eventually, I do expect that. We don’t know for sure how that impact will be felt. In the case of quantum computing, the best idea we currently have — and it’s an old idea, which goes back over 40 years to Richard Feynman — is that we can use quantum computers to understand more deeply how quantum systems behave.
Physicists like us understand that that’s interesting, but it’s also important because it can enable the discovery of new types of materials with useful properties, new types of chemical compounds, perhaps including pharmaceuticals and so on. And all that eventually does affect people’s everyday lives. And with quantum measurement as well, I think quantum technology is really going to touch everything in science eventually.
Let’s say in biology and medicine, we’d like to be able to observe what’s going on inside cells, non-invasively and with higher sensitivity. And that’s going to be important for therapies eventually, and it’ll also be important for understanding biological science more deeply.
LEVIN: There’s also a place for quantum teleportation in understanding the fundamental nature of gravity, which I know has been a central area of your research. How could entanglement possibly play a role in things so big and lumbering as black holes?
PRESKILL: For me, this is one of the most exciting things about quantum information, it’s that it’s giving us fresh ways of thinking about other fundamental questions, including in condensed matter physics, where we’re trying to understand highly entangled states of quantum matter, and in gravitational physics.
This story goes back a long way to 1935 when two famous papers appeared in the Physical Review. One of them, by Einstein and [Nathan] Rosen, was about the observation that we can find solutions in general relativity to Einstein’s equations, which describes space-time, in which there’s a wormhole in space. This wasn’t understood so well at the time, but really, the solution describes two black holes, which have a shared interior — a kind of wormhole connecting the inside of these two black holes.
And the paper by Einstein, [Boris] Podolsky and Rosen was about quantum entanglement and the peculiar way in which it allows systems to be correlated with one another in a way that we can’t describe in terms of classical information.
And what we’ve come to appreciate in the last 10 years: These two phenomena, quantum entanglement and wormholes in space, are closely related to one another. In fact, they can be viewed as two ways of describing the same thing. This is a common thing in physics and very empowering. If we have two different ways to describe the same phenomenon, which look very different from one another, but describe exactly the same physics, that can empower us to get a deeper understanding.
And so, what we appreciate now, and which we can say pretty explicitly in the version of quantum gravity that we understand best, is that if two black holes become very highly entangled with one another, they will be connected by a wormhole in space.
Alice could have her black hole, and Bob could have his, and if they’re entangled with one another, it means that Alice and Bob could both jump into their black holes. And then they could meet, and perhaps have a relationship for a while, although they’d be doomed, like Romeo and Juliet, to hit the singularity and be destroyed. But we can make it even more fun, and this is where teleportation comes in.
We can make a wormhole in space, under just the right conditions, traversable. The original wormhole that was originally described by Einstein and Rosen is an example of a non-traversable wormhole. That means you can’t jump in one end and come out on the other end. But what we’ve come to appreciate is that it’s actually possible in quantum theory to send in a negative energy pulse into a black hole. When you normally send matter into a black hole, it makes its event horizon move outward a little bit, that negative energy pulse can make it move inward a little bit. And that’s just what we need to be able for Alice to throw a bit or a qubit into her black hole and for it to come out at Bob’s end.
There’s an alternative way of describing this, which is this is really a form of quantum teleportation.
So I think that’s really fun, because it suggests that gravitational intuition can help us to understand the behavior of very complex quantum systems which otherwise would seem very nonintuitive.
LEVIN: It’s an absolutely awesome and fascinating turn to delve that deep into the quantum, to try to understand the large-scale phenomena, like the very existence of black holes or their survival.
And I’m going to sneak in one question about the evaporation of black holes, and how quantum teleportation could be relevant in understanding how, if Alice jumps into her black hole, her information may not ultimately be lost, and that quantum teleportation might be a way for us to recover what happened to Alice after she jumped into the black hole.
PRESKILL: Well, I knew when I got together with Janna Levin, we’d eventually be talking about black holes.
LEVIN: [laughing] I can turn any conversation into a conversation about black holes.
PRESKILL: No surprise there.
Actually, I think what I just described does give us insight into the process by which information escapes from black holes, which we believe that it does. The laws of physics don’t allow information to be destroyed, even when it falls into black holes and the black holes evaporate. It just gets scrambled up into some form that’s exceedingly hard to read. There’s some kind of violation of locality. This is the most, or one of the most, fundamental principles in physics. We referred to it earlier — that information can’t travel faster than the speed of light.
But, in some sense, to get out of a black hole, by definition information is traveling faster than light. Light is trapped inside, information gets out. And what that indicates is that the notion of causality — the way we usually think about it, that there’s a speed limit on how fast information can travel — is not rigorously true under all circumstances. That principle can be violated.
And space-time itself may not really be a fundamental notion. Rather, it’s an emergent property of some complex quantum system in which things are highly entangled.
So how is it that we think, under normal circumstances, that this notion of causality seems to be so rigorously satisfied? Well, I think we have an answer for that, and it’s quite interesting that it connects with quantum computing.
We think it’s possible to violate causality, to send information faster than light. But in order to do so requires a quantum computation of the sort that you might do on a quantum computer, which is so complex and so powerful that we’ll never be able to do it in practice.
So, we should be able to rip apart the space in between me in California and you, Janna, in New York. In principle, we can. In practice, it’s so exceedingly hard to do it, it would require such a powerful computation, that no one will ever succeed.
LEVIN: Remarkable. Now, John, you’ve spent a lot of your life trying to understand some of the most elusive and challenging concepts in quantum theory. What is it about the study of theoretical physics and quantum teleportation that brings you joy?
PRESKILL: Well, I’m pretty easy to entertain, so lots of things bring me joy. But both questions and answers can bring one joy. Ideas that, you know, you’ve never heard before and you realize are deep and fascinating can bring joy. So when I first appreciated that we can theoretically — and I think eventually in practice — build quantum computers that are so powerful that they’ll be able to solve problems that we’d never be able to solve if this were a classical world, that was kind of one of the happiest moments, to encounter such a deep and interesting idea. And thinking about that eventually led me to change the direction of my own research.
LEVIN: It’s such beautiful stuff. We’ve been speaking with Caltech theoretical physicist John Preskill about the incredible nature and potential applications of quantum teleportation. John, thank you so much for being with us today.
PRESKILL: I had a great time, Janna. Thank you.
LEVIN: Me too. It’s always fun to talk. ’Til soon.
[Theme plays]
LEVIN: “The Joy of Why” is a podcast from Quanta Magazine, an editorially independent publication supported by the Simons Foundation. Funding decisions by the Simons Foundation have no influence on the selection of topics, guests or other editorial decisions in this podcast or in Quanta Magazine.
“The Joy of Why” is produced by PRX Productions. The production team is Caitlin Faulds, Livia Brock, Genevieve Sponsler and Merritt Jacob. The executive producer of PRX Productions is Jocelyn Gonzales. Morgan Church and Edwin Ochoa provided additional assistance. From Quanta Magazine, John Rennie and Thomas Lin provided editorial guidance, with support from Matt Carlstrom, Samuel Velasco, Nona Griffin, Arleen Santana and Madison Goldberg.
Our theme music is from APM Music. Julian Lin came up with the podcast name. The episode art is by Peter Greenwood and our logo is by Jaki King and Kristina Armitage. Special thanks to the Columbia Journalism School and Bert Odom-Reed at the Cornell Broadcast Studios.
I’m your host, Janna Levin. If you have any questions or comments for us, please email us at [email protected]. Thanks for listening.
- SEO Powered Content & PR Distribution. Get Amplified Today.
- PlatoData.Network Vertical Generative Ai. Empower Yourself. Access Here.
- PlatoAiStream. Web3 Intelligence. Knowledge Amplified. Access Here.
- PlatoESG. Carbon, CleanTech, Energy, Environment, Solar, Waste Management. Access Here.
- PlatoHealth. Biotech and Clinical Trials Intelligence. Access Here.
- Source: https://www.quantamagazine.org/what-is-quantum-teleportation-20240314/