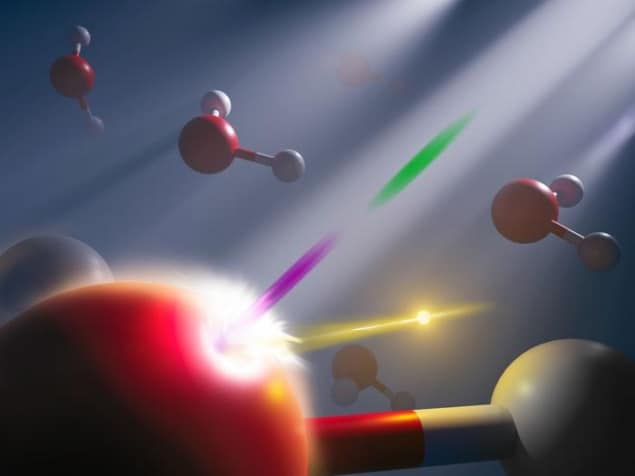
Scientists can now follow the movement of electrons and the ionization of molecules in real time thanks to a new attosecond X-ray spectroscopy technique. Like stop-motion photography, the technique effectively “freezes” the atomic nucleus in place, meaning that its motion does not skew the results of measurements on the electrons whizzing around it. According to the technique’s developers, it could be used not only to probe the structure of molecules, but also to track the birth and evolution of reactive species that form via ionizing radiation.
“The chemical reactions induced by radiation that we want to study are the result of the electronic response of the target that happens on the attosecond timescale (10-18 seconds),” explains Linda Young, a physicist at Argonne National Laboratory and the University of Chicago, US, who co-led the research together with Robin Santra of the Deutsches Elektronen-Synchrotron (DESY) and the University of Hamburg in Germany and Xiaosong Li of the University of Washington, US. “Until now, radiation chemists could only resolve events at the picosecond timescale (10-12 seconds), which is a million times slower than an attosecond. It’s kind of like saying ‘I was born and then I died.’ You’d like to know what happens in between. That’s what we are now able to do.”
Pump and probe
The new technique works as follows. First, the researchers apply an attosecond X-ray pulse with a photon energy of 250 electron volts (eV) to a sample – of water, in this case, though the team say the technique could work with a wide range of condensed-matter systems. This initial “pump” pulse excites electrons from the water molecule’s outer (valence) orbitals, which are responsible for molecular bonding and chemical reactions. These orbitals are further from the atomic nucleus, and they have much lower binding energies than the inner “core” orbitals: around 10-40 eV compared to about 500 eV. This makes it possible to ionize them – a process known as valence ionization – without affecting the rest of the molecule.
Around 600 attoseconds after the valence ionization, the researchers fire a second attosecond pulse – the probe pulse – at the sample, with an energy of around 500 eV. “The short time delay between the pump and probe pulses is one of the reasons why the hydrogen atoms themselves do not have time to move and are like ‘frozen’,” Young explains. “This means their movement does not affect the measurement results.”
When the probe pulse interacts with the holes (vacancies) left behind in the valence orbitals following valence ionization, the pulse’s energy distribution changes. By reflecting the pulse from a grating that disperses this energy distribution onto a two-dimensional detector, the researchers obtain what Young calls a spectral “snapshot” or “fingerprint” of electrons occupying the valence orbitals.
Finding flaws in earlier results
By observing the motion of the X-ray-energized electrons as they move into excited states, the researchers uncovered flaws in the interpretation of earlier X-ray spectroscopy measurements on water. These earlier experiments produced X-ray signals that appeared to stem from different structural shapes, or “motifs,” in the dynamics of water or hydrogen atoms, but Santra says the new study shows this is not the case.

“In principle, one could have thought that the timing precision of this type of experiment is limited by the lifetime (which is around a couple of femtoseconds, or 10-15 seconds) of the X-ray-excited electronic quantum states produced,” he tells Physics World. “Through quantum-mechanical calculations, however, we showed that the observed signal is confined to less than a femtosecond. This is the reason why we were able to show that X-ray spectroscopy measurements on the structure of liquid water had been previously misinterpreted: unlike these earlier measurements, ours were not affected by moving hydrogen atoms.”
Experimental goals and challenges
The researchers’ initial goal was to understand the origin of reactive species created when X-rays and other forms of ionizing radiation impinge on matter. These reactive species form on an attosecond time scale following ionization, and they play important roles in biomedical and nuclear science as well as chemistry.
Attosecond electron pulses are claimed as shortest ever
One of the challenges they encountered was that the X-ray beamline they used – ChemRIXS, part of the Linac Coherent Light Source at the SLAC National Accelerator Laboratory in Menlo Park, California – had to be completely reconfigured to perform all-X-ray attosecond transient absorption spectroscopy. This powerful new technique makes it possible to study processes on extremely short time scales.
The researchers now plan to extend their studies from pure water to more complex liquids. “Here, the different molecular constituents can act as traps for the freed electrons and produce new reactive species,” Young says.
They report their present work in Science.
- SEO Powered Content & PR Distribution. Get Amplified Today.
- PlatoData.Network Vertical Generative Ai. Empower Yourself. Access Here.
- PlatoAiStream. Web3 Intelligence. Knowledge Amplified. Access Here.
- PlatoESG. Carbon, CleanTech, Energy, Environment, Solar, Waste Management. Access Here.
- PlatoHealth. Biotech and Clinical Trials Intelligence. Access Here.
- Source: https://physicsworld.com/a/new-attosecond-x-ray-spectroscopy-technique-freezes-atomic-nuclei-in-place/